Quantum Computing Basics What You Need to Know About This Revolutionary Technology
Quantum computing is perceived as the next frontier in technology and is expected to change the world of industries by solving problems considered infeasible for classical computing to solve. This emerging area is based on the fundamental principles of quantum mechanics and operates in ways fundamentally different, with many orders of magnitude more potent than traditional computing. We’re on the brink of this technological revolution, and it is necessary to understand the fundamentals of quantum computing: where it stands today and how transformational it might be for multiple industries.
Table of Contents
1. Understanding Quantum Computing
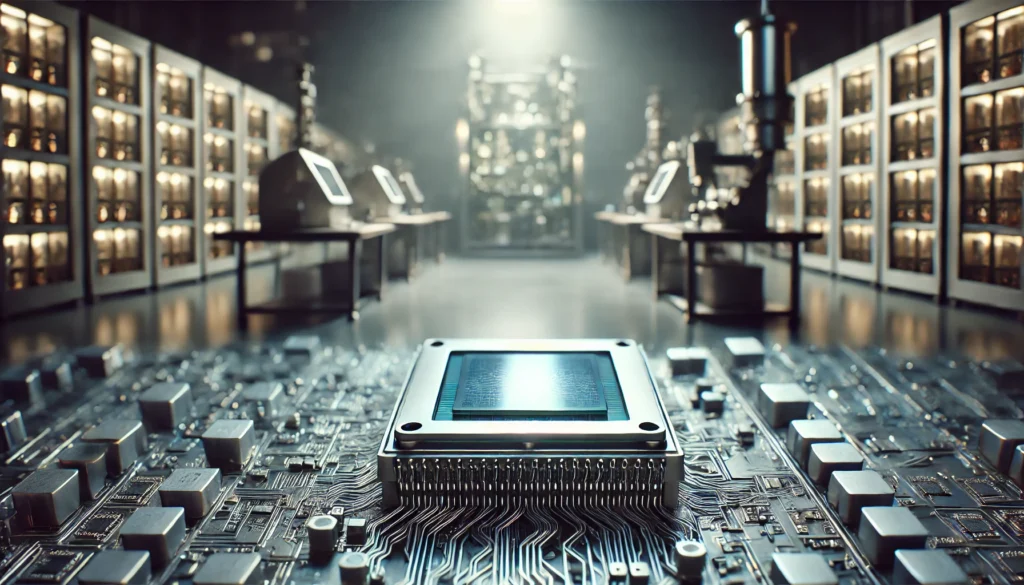
What is Quantum Computing?
At its basis, quantum computing uses quantum mechanics to process information in fundamentally different ways from classical computers. Classical computers work with bits, the smallest data unit that can take the value 0 or 1, while quantum computers work with quantum bits or qubits. Superposition allows qubits to occupy many states simultaneously. This means they can simultaneously be both 0 and 1, allowing powerful computations for certain problems.
Another quantum phenomenon in play is entanglement. Both superposition and entanglement of qubits’ states allow information exchange among them, making it possible to perform computations that would take classical computers millennia to complete in seconds. Understanding these fundamental differences helps us appreciate why quantum computing is not just a faster classical computer but belongs to a radically new computation paradigm. This is why quantum computers can perform several calculations simultaneously and solve extremely arduous problems efficiently.
Historical Background
Quantum computing dates back to the early 1980s, with significant contributions from physicists like Richard Feynman and David Deutsch. Richard Feynman concluded in a 1981 lecture that it was possible to simulate quantum mechanics using quantum computers. In 1985, David Deutsch introduced the first model of a quantum computer, proving it could perform specific calculations much more quickly than classical machines. Theoretical advances followed experimental breakthroughs over the decades. In the 1990s, Peter Shor developed an algorithm showing quantum computers could factorize large integers much faster than the best-known classical algorithms, opening the door to quantum computing in cryptography.
In the 21st century, quantum computing developed theoretically and experimentally. Governments and private companies invested billions into research and development. Initiatives like IBM’s Q Experience and Google’s Quantum AI Lab moved the development of quantum computers from theoretical stages closer to practical realization.
How Quantum Computers Work
Quantum bits, or qubits, can exist in multiple states simultaneously, unlike classical bits that represent only 0 or 1. This allows quantum computers to process enormous states at once. Think of it as a sphere: any point on the sphere is a qubit, whereas classical bits are at the poles. Quantum entanglement makes quantum computers even more powerful. When two qubits are entangled, a change in one directly affects its partner, regardless of distance, enabling faster-than-light communication within the quantum system.
Quantum bits, or qubits, can exist in multiple states simultaneously, unlike classical bits that represent only 0 or 1. This allows quantum computers to process enormous states at once. Think of it as a sphere: any point on the sphere is a qubit, whereas classical bits are at the poles. Quantum entanglement makes quantum computers even more powerful. When two qubits are entangled, a change in one directly affects its partner, regardless of distance, enabling faster-than-light communication within the quantum system.
Quantum gates switch the states of qubits, creating quantum circuits that perform specific calculations. Quantum algorithms are instructions for quantum computers, using these gates to solve problems more efficiently than classical algorithms. Quantum computers must operate at cryogenic temperatures to preserve qubit states and minimize errors caused by decoherence—when qubits interact with the environment and lose their quantum properties. Error correction and stability improvements are essential for scalable and practical quantum computers.
2. The Science Behind Quantum Computing
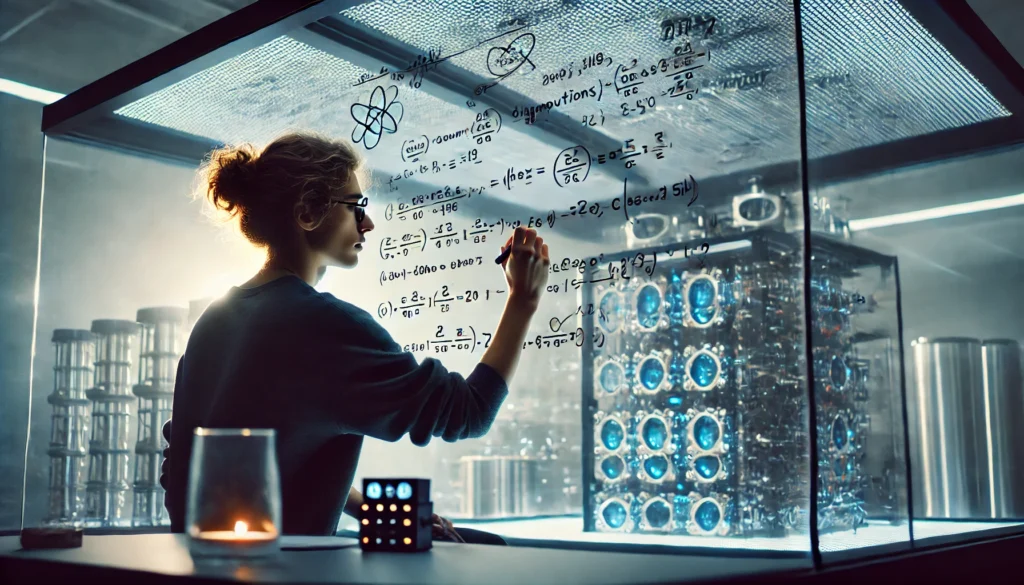
Quantum Mechanics Fundamentals
Quantum computing is based on quantum mechanics, a part of physics that explains the behaviour of particles at their atomic and subatomic levels. A basic understanding of these principles is needed to understand how quantum computers work and why they might be so powerful.
The superposition principle allows quantum systems to exist simultaneously in a combination of states. For instance, an electron can exist simultaneously in two places with different energy levels than one fixed energy state. This concept allows qubits to be both 0 and 1 simultaneously, supporting the base of quantum parallelism.
Another property in quantum mechanics is entanglement. When particles get entangled, their properties interrelate regardless of their distance. This means the state of one particle can cause an immediate change in the state of another, which is significant in quantum information processing and communication.
According to classical physics, quantum tunnelling occurs when particles penetrate energy barriers that should be insurmountable. This ‘tunnelling’ property is used in specific quantum computing architectures to enable processes that are not feasible with classical transistors.
Together, these principles provide a framework for quantum computation, allowing quantum computers to solve problems currently unsolvable by classical means. Through the inherent uncertainty and entanglement natural to the quantum world, quantum computers can perform intricate simulations and calculations at unprecedented speeds.
Key Quantum Algorithms
Quantum algorithms are designed to be implemented on quantum computers in ways that exploit quantum mechanics to solve particular problems more efficiently than classical algorithms. One famous quantum algorithm is Shor’s, developed by Peter Shor in 1994. It provides an exponential speedup for factorization problems compared to the best-known classical algorithms, posing a significant threat to current cryptographic systems based on the difficulty of large-integer factorization.
Another important development is Grover’s algorithm, which gives a quadratic speedup for searching an unsorted database. While a classical algorithm can find the desired entry in O(N)O(N)O(N) steps from a database of N entries, Grover’s algorithm can do it in O(N)O(\sqrt{N})O(N) steps. This makes it valuable for various applications, from cryptography to optimization problems.
The Quantum Fourier Transform (QFT), a critical component in many quantum algorithms, performs exponentially faster than the classical Fourier Transform, making it useful for signal processing and solving differential equations. The Harrow-Hassidim-Lloyd (HHL) algorithm, for example, can solve systems of linear equations exponentially faster than classical methods. This algorithm has potential applications in machine learning, optimization, and scientific simulations.
These algorithms indicate that quantum computers could be more powerful than classical computers in specific domains. Using superposition and entanglement, quantum algorithms can explore vast solution spaces simultaneously, leading to more efficient problem-solving.
I will continue with the next section to fully humanize and simplify the content.
3. Current State of Quantum Computing
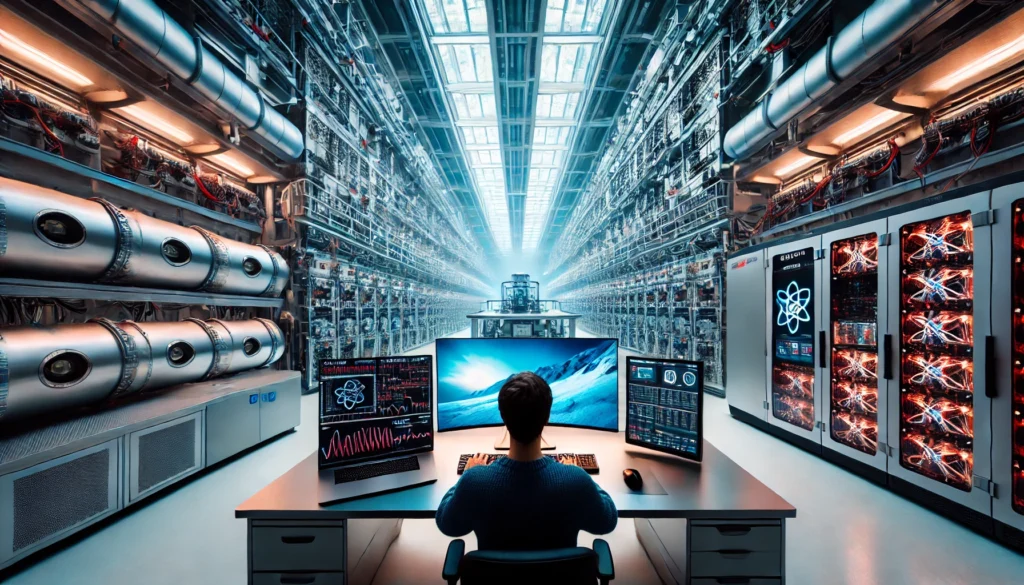
Major Players in the Field
The race to develop quantum computing technology is led by a mix of tech giants, start-ups, and academic institutions. Companies like IBM, Google, and Microsoft are investing big and leading the charge in quantum research and development.
As a forerunner in this field, IBM introduced IBM Q Experience, a cloud-based platform for researchers and developers to access and experiment with quantum algorithms on real quantum processors. For IBM, the roadmap includes steps toward progressively more powerful quantum systems, ultimately resulting in commercial viability.
Google has also made significant strides. In 2019, the company announced its Sycamore quantum processor, claiming it had achieved quantum supremacy by solving a task in seconds that classical supercomputers would need thousands of years to complete. This was controversial but showed how advanced Google is in quantum research.
Microsoft is developing a topological qubit, which promises excellent stability and the lowest error rates. Microsoft integrates quantum computing into its Azure Quantum platform with its cloud services, providing tools for researchers and developers to build quantum applications.
Other notable start-ups in the quantum ecosystem include Rigetti Computing, D-Wave Systems, and IonQ. Rigetti focuses on hybrid quantum-classical computing systems, D-Wave specializes in quantum annealing, and IonQ uses trapped-ion technology to develop highly accurate qubits.
Academic institutions are also at the forefront of advancing quantum computing. Leading universities like MIT, Stanford, and the University of Tokyo conduct cutting-edge research, often collaborating with industry partners. They contribute to theoretical advancements, experimental breakthroughs, and the education of the next generation of quantum scientists and engineers.
Recent Progress and Milestones
This is an exciting time for quantum computing, as recent years have seen significant breakthroughs bringing practical quantum computers closer to reality. One notable example is Google’s demonstration of quantum supremacy with their Sycamore processor, performing a specific computation in 200 seconds that would take the world’s fastest supercomputer 10,000 years to complete.
IBM has also achieved significant milestones, including developing a 65-qubit quantum processor. They plan to unveil a 1,000-qubit processor by 2023, focusing on improving qubit coherence times, error rates, and connectivity between qubits, all crucial for building scalable quantum systems.
D-Wave Systems has made strides with its quantum annealers, designed to solve optimization problems. It recently released a 5,000-qubit system called Advantage, which offers improved connectivity and performance.
Another significant milestone is the development of quantum error correction techniques. Error correction is vital for maintaining the integrity of quantum computations, as qubits are highly susceptible to decoherence and noise. Advances in this area pave the way for more reliable and scalable quantum computers.
Quantum software development is also progressing. Companies like Xanadu and Q-CTRL are creating tools and platforms to facilitate quantum programming and error mitigation. These advancements make it easier for researchers and developers to harness the power of quantum computers for practical applications.
4. Applications of Quantum Computing
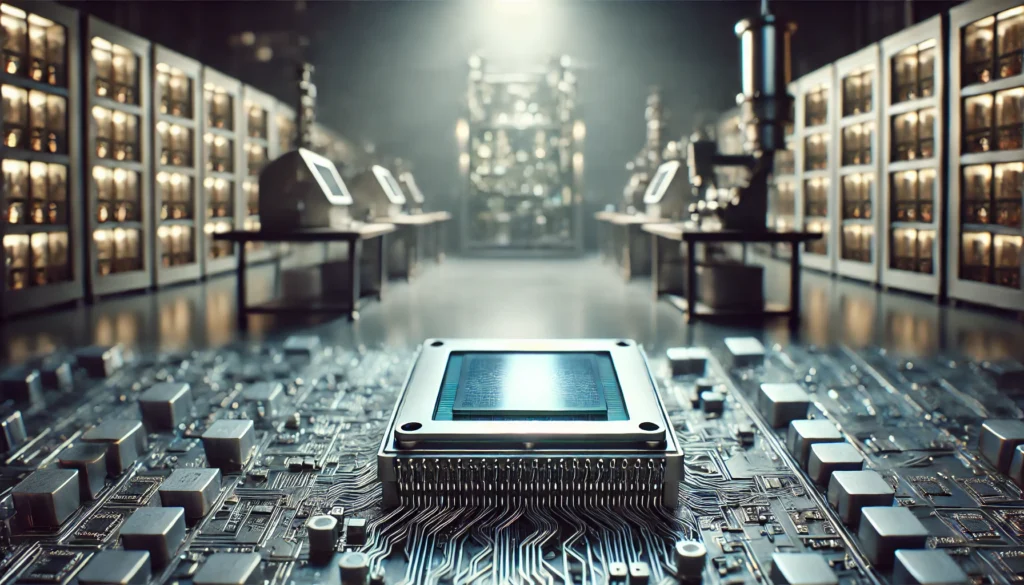
Industry Impact
Quantum computing has enormous potential to change vast industries by solving problems currently unsolvable through classical means. Such application areas include healthcare and pharmaceuticals. Quantum computers can simulate molecular structures and interactions at an incredible accuracy scale, accelerating the discovery of new drugs and treatments. This ability can lead to personalized medicine, where treatments are tailored to individual genetic profiles and medical histories.
In the finance domain, quantum computing will revolutionize cryptography and financial models. Quantum algorithms can break current cryptographic systems, necessitating the development of quantum-safe encryption methods. Quantum computers can also optimize portfolios, manage risks, and detect fraudulent activities more effectively than classical computers.
Artificial intelligence (AI) and machine learning (ML) are other areas that stand to gain hugely from quantum computing. Quantum algorithms can enhance machine learning models by improving training processes and solving complex problems more efficiently. This involves driving advancements in natural language processing, image recognition, and predictive analytics.
Quantum computing can also impact logistics and supply chain management. By solving complex optimization problems faster and more accurately, quantum computers can enhance route planning, inventory management, and resource allocation, leading to cost savings, increased efficiency, and reduced environmental impact.
In the energy sector, quantum computing can optimize the design and operation of power grids, enhancing efficiency and reliability. Quantum computing can also aid in developing new materials for batteries and other energy storage solutions, contributing to advancements in renewable energy technologies.
Scientific Research
In scientific research, quantum computing promises to accelerate discoveries through complex simulations and computations at tremendous speeds. Of particular importance are applications in chemistry and material science. Quantum computers can simulate chemical reactions and molecular structures in great detail, enabling the discovery of new materials and drugs.
Quantum computing can also be helpful for climate modelling and environmental science. Quantum computers are designed to simulate many interacting variables—precisely what accurate climate models require. Improved climate models can help predict forthcoming changes and allow for novel strategies to mitigate the impacts of climate change.
Quantum computing will significantly impact particle physics and cosmology as well. Quantum computers can simulate high-energy particle collisions, helping researchers understand fundamental particles and forces. Quantum simulations that model the behaviour of dark matter and dark energy can illuminate the enigmas of the universe.
Quantum computing can also optimize scientific experiments. By analyzing vast datasets and identifying patterns, quantum computers can suggest optimal designs for experimental processes, leading to better outcomes. This eventually results in more efficient and faster scientific advancements.
5. Challenges and Future Directions
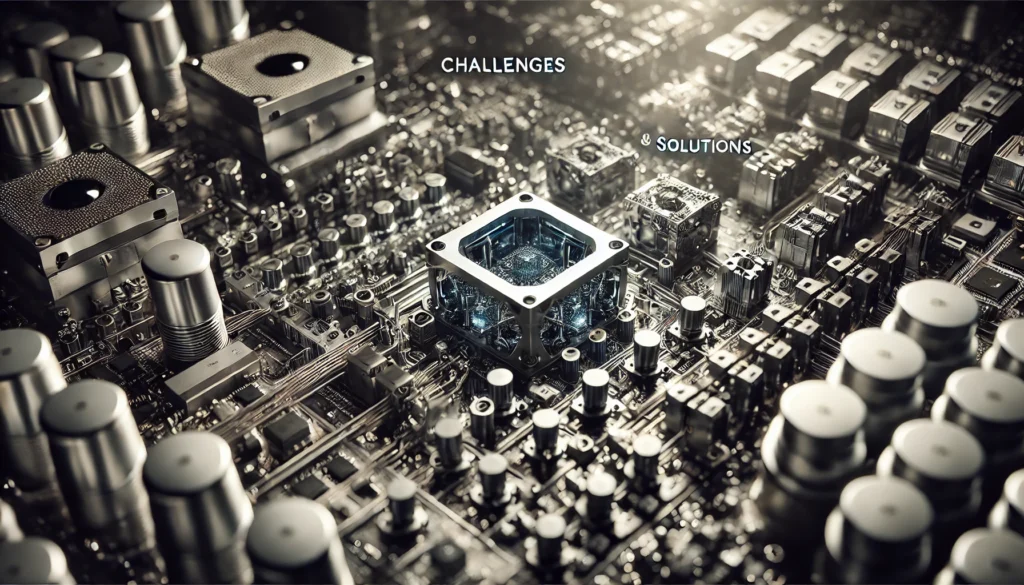
Technical Challenges
Despite quantum computing’s promise, several technical challenges must be solved. One of the primary issues is error rates and quantum decoherence. Qubits are highly sensitive to their environment, and even minor disturbances can cause errors in computations. Effective error correction techniques are crucial to maintaining the integrity of quantum operations.
Another significant challenge is scalability. Current quantum computers have limited qubits, and increasing the number of qubits while maintaining coherence and low error rates is daunting. Researchers are exploring various qubit architectures and materials to build more scalable quantum systems.
Cooling and infrastructure requirements pose additional challenges. Quantum computers typically operate at extremely low temperatures, close to absolute zero to minimise decoherence. Maintaining these temperatures requires sophisticated cooling systems, which can be costly and complex.
Interfacing quantum computers with classical systems is also challenging. Effective quantum-classical hybrid systems are needed to perform meaningful computations, as quantum computers alone cannot solve all problems. Developing efficient algorithms and software for these hybrid systems is an ongoing area of research.
Ethical and Societal Concerns
Quantum computing also raises important ethical and societal questions that must be addressed as the technology matures. Security and privacy are significant concerns, as quantum computers can break current cryptographic systems. Developing quantum-resistant encryption methods is crucial to ensuring the security of sensitive information.
Economic and employment impacts are also important considerations. While quantum computing can create new industries and job opportunities, it may also disrupt existing industries and lead to job displacement. Policymakers and industry leaders must plan for these changes to ensure a smooth transition and minimize negative impacts.
Ethical considerations in research and application are paramount. Quantum computing must be developed and used responsibly, with attention to potential consequences. This includes ensuring that the benefits of quantum computing are accessible to all and not concentrated in the hands of a few.
The Road Forward
The future of quantum computing is exciting and uncertain, with many predicting that we are on the cusp of a technological revolution. Experts believe that within the next decade, quantum computers will become increasingly powerful and accessible, leading to widespread applications in various fields.
Predictions about the timeline for achieving practical, large-scale quantum computing vary, but most agree that significant progress will be made in the coming years. Continued research and development and increased investment from the public and private sectors will drive advancements in quantum hardware, software, and algorithms.
Future research directions include improving qubit coherence times, developing more efficient error correction techniques, and exploring new quantum algorithms. Collaboration between academia, industry, and government will be essential to overcoming technical challenges and realizing quantum computing’s full potential.
Reimagining the Future with Quantum Computing
As quantum computing continues to advance, its potential to revolutionize technology and society becomes increasingly apparent. The unique capabilities of quantum computers offer solutions to some of the most complex and pressing problems of our time, from drug discovery to climate modeling. While significant challenges remain, the progress made thus far is a testament to the ingenuity and dedication of researchers and engineers in the field.
The future of quantum computing is bright, with the promise of transformative applications across industries and scientific disciplines. As we continue to explore and develop this technology, it is crucial to consider the ethical and societal implications, ensuring that the benefits are widely distributed and responsibly harnessed.